Advancing our understanding of how the brain works
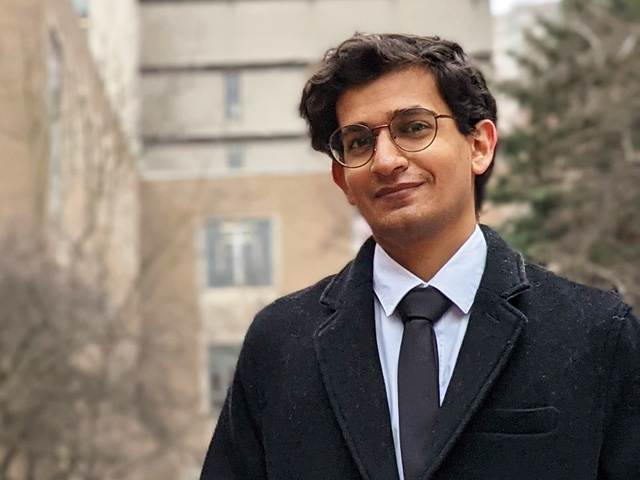
Faheem Mosam
Though once under passionate dispute, there are countless scientific theories that have now passed into fact: that the sun is at the centre of our universe, that certain diseases are caused by microorganisms invisible to the eye, that human activities have led to climate change, to name just a few.
The brain criticality hypothesis has not achieved this kind of consensus. Not only has it yet to make its way into popular understanding, it remains under debate in the scientific community.
In simple terms, criticality is a theory with the potential to explain brain activity. It posits that the brain exists in a critical state that lies between order and chaos, a balanced “sweet spot” of regularity and randomness. Brains require a degree of order to function, but too much order leads to stasis, a kind of stoppage in function. At the other end of the spectrum, chaos or too much randomness also undermines proper function. Criticality—neither too much order nor disorder—is assumed to be an optimal mode for brain functioning, as it makes it possible for the system to be flexible and adapt.
But there is still much to understand about the brain criticality hypothesis, which is why Department of Physics master’s student Faheem Mosam was interested in contributing to the debate. Along with co-authors Professors Eric De Giuli, also of the Department of Physics, and Diego Vidaurre of Oxford University’s Department of Psychiatry, Mosam recently published in Physical Review E.
“Our research defined a temperature parameter for a certain way of modelling dynamic behaviour called Hidden Markov Models,” says Mosam. “This temperature parameter behaves like a scale that takes the system between order (cold) and chaos (hot). Similar to how molten glass has no defined structure but will take one on during a phase transition as it cools down, a network can take on structure as it transitions from hot to cold in our temperature parameter.”
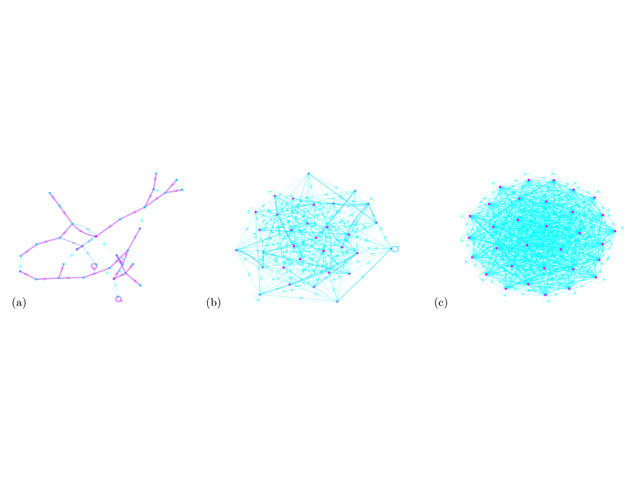
Representative networks at various temperature parameters.
To ensure the model is accurate and applicable to biological systems, the team analyzed data from 820 human subjects who lay still in an fMRI machine at rest. After building the Markov Models of the subjects, each could have their temperature measured.
“What we found was that the human data supported the brain criticality hypothesis, because the temperatures were all around the theoretically predicted critical value,” says Mosam. “Most research done on brain criticality has looked at the neuronal level, whereas this work looked at the level of whole-brain activity. Showing that whole-brain activity of humans at rest lies in the critical region was a very exciting contribution to a greater understanding of the brain and criticality in biological systems.”
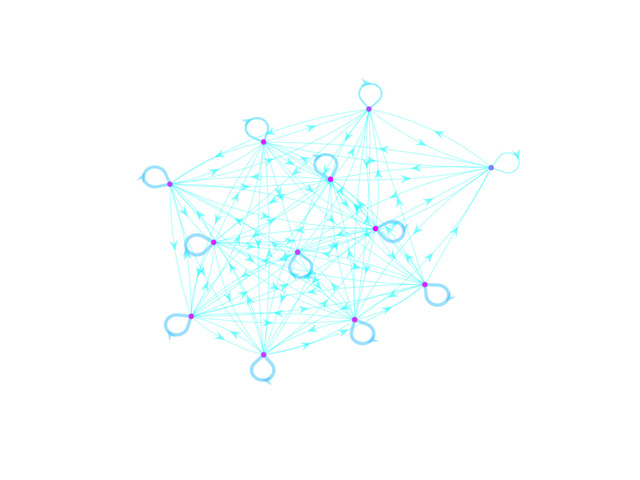
Representative network for the Hidden Markov model applied to neural data.
The team’s next step is to define a coupling parameter so that transitions between two neighbouring states can have a scale that investigates dependency of the system transitioning between those states. They also hope to apply this model to data from brains in different states of consciousness, such as under anesthesia or performing a task, to see if there is any correlation between certain levels of criticality and states of consciousness. Are brains closer to the side of chaos when performing a task? Are they more ordered under anesthesia? Such analysis could give greater insight into the relationship between criticality in the brain and consciousness.
Beginning this work as his fourth-year thesis project, Mosam has found it incredibly rewarding to have it grow and lead to a publication.
“I am especially grateful to have been able to interact with researchers around the world and to work with Dr. Vidaurre,” says Mosam. “When I started, I had no idea this would become an interdisciplinary collaboration.”
The team is grateful to Anna Stavyska for her preliminary work on this project. In addition, Eric De Giuli is supported by an NSERC Discovery Grant and Diego Vidaurre is supported by a Novo Nordisk Emerging Investigator Award and by the European Research Council.