New Insight into Cell Mechanics Boosts Cancer-Killing Drug Therapy
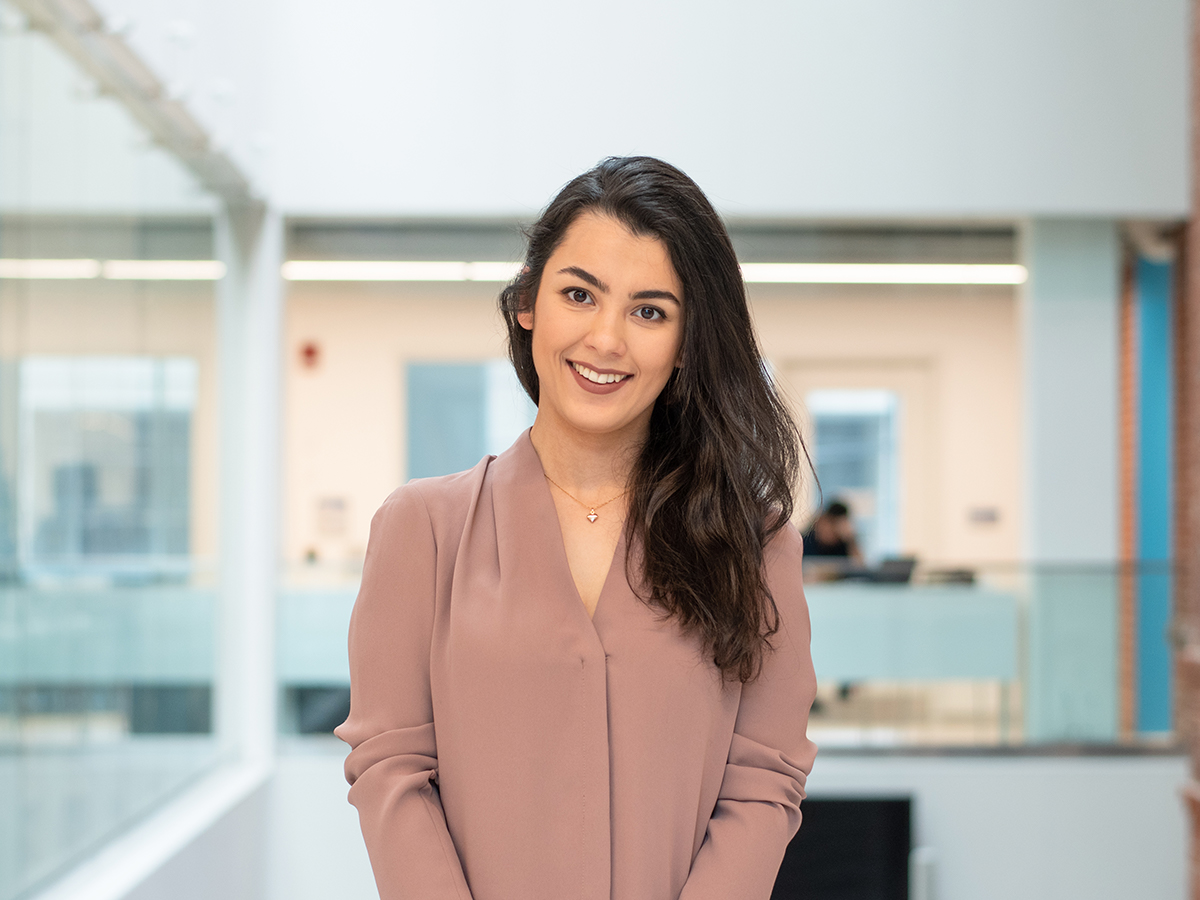
Long considered the cure that’s worse than the disease, chemotherapy still strikes terror into the hearts of cancer patients. With a reputation for bringing hair loss, vomiting and other grievous side effects, traditional chemo treatments resemble what biologist Costin Antonescu (external link) calls a “sledgehammer approach” – damaging healthy cells in the process of killing cancerous ones.
Fortunately, oncology is advancing rapidly, and work is underway to develop precision medicine. Rather than distributing chemo drugs uniformly throughout the body, targeted delivery puts drugs exactly where they’re needed – at the tumour site. Ultrasound and microbubbles is one technology capable of penetrating cancer cell walls and releasing chemo drugs on command. But to leverage its power, scientists must first understand how the cell allows drugs to enter inside its walls.
Molecular Science PhD Farnaz Fekri has been exploring this biology-physics interface since her undergraduate days. Now, after a six-year interdisciplinary collaboration supervised by Antonescu and Physics professor, Raffi Karshafian, Fekri and her co-authors published their results (external link) in Scientific Reports, a Nature-family journal.
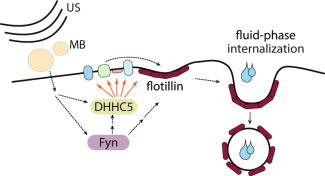
Model of USMB-triggered enhancement of flotillin-dependent fluid-phase internalization.
Manipulating Cellular Mechanisms for Better Drug Delivery
While cancer-fighting drugs may be effective in themselves, various factors can limit their impact – especially when administered through traditional methods such as injection or pills. Fekri explains: “The size of the tumour, irregular blood flow, stomach acids – these can all impede drugs from reaching tumours effectively. But another important barrier is the cell’s own membrane.”
Surrounding each cell is a bag-like, plasma membrane that controls what enters inside. Endocytosis is one way that nutrients or other molecules pass through. Some types of endocytosis require molecules to bind to a receptor before entering. Another type, fluid-phase endocytosis, has no such requirement. Instead, a section of cell membrane gulps up fluid outside the cell, forms a sac-like vesicle, and internalizes the fluid into the cell.
What if there were a way to boost this process? Fekri explains the implications: “If we can better understand how the endocytic mechanism works, we can then manipulate it — not for nutrient uptake, but to force cancer cells to take up chemo drugs in a targeted, localized way.”
Ultrasound Microbubbles Stimulate Cellular Processes
Ultrasound and microbubbles (USMB) comprises the physics arm of Fekri’s research study. Clinical microbubbles are microscopic balls of gas enclosed in a lipid shell. Ultrasound beams penetrate not air, but fluid-filled spaces – such as blood – and can focus narrowly on a small region of tissue, such as tumour cells.
When microbubbles are exposed to varying pressures, they can expand, contract, oscillate and vibrate – up to two million times per second. What if microbubbles were placed next to cancer cell membranes and stimulated by an ultrasonic pulse? How would cells respond to the disturbances caused by the excited microbubbles?
Fekri and her colleagues discovered that after sensing the nearby effects of USMB, the cells accelerated their own natural process of flotillin-dependent endocytosis. The results were large increases in both drug uptake and, in turn, cancer cell death.
Fekri and her colleagues eventually identified flotillin other protein signals and switches involved in fluid-phase endocytosis. “There’s still a lot we don’t understand, but we did discover some of the key players and how these proteins talk to each other to activate endocytosis. It’s an early step in controlling cellular activity to fight cancer.”
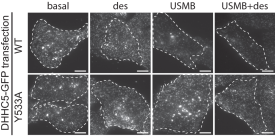
Expression of a phosphorylation-defective mutant of DHHC5 impairs flotillin internalization elicited by USMB treatment.
Looking Ahead: From Bench to Bedside
After completing six years of research, Fekri is excited about the future of USMB in precision medicine. The hope is to eventually incorporate chemo drugs into microbubbles and use ultrasound to release it at a specific site in the body.
“As the field evolves, we’ll be able to move away from limited, conventional drug administration methods. With better understanding of cell mechanics, we can not only design new drugs, but ensure that the drugs reach the actual disease site and kill cancer cells more effectively, while sparing healthy cells.”
It will still be years before the technology can finally be used on patients, but the prospects are promising. Karshathian concludes: “We’re not the first to discover that USMB treatment boosts drug delivery, but by understanding the mechanical processes involved, we’re adding another piece of the puzzle in this fight against cancer.”
Fekri’s work was funded by grants and awards from NSERC, Canadian Institute of Health Research and the Ontario Ministry of Research, Innovation and Science.