Rethinking Plastics: The Promise and Challenges of Starch-Based Bioplastics for Sustainable Packaging
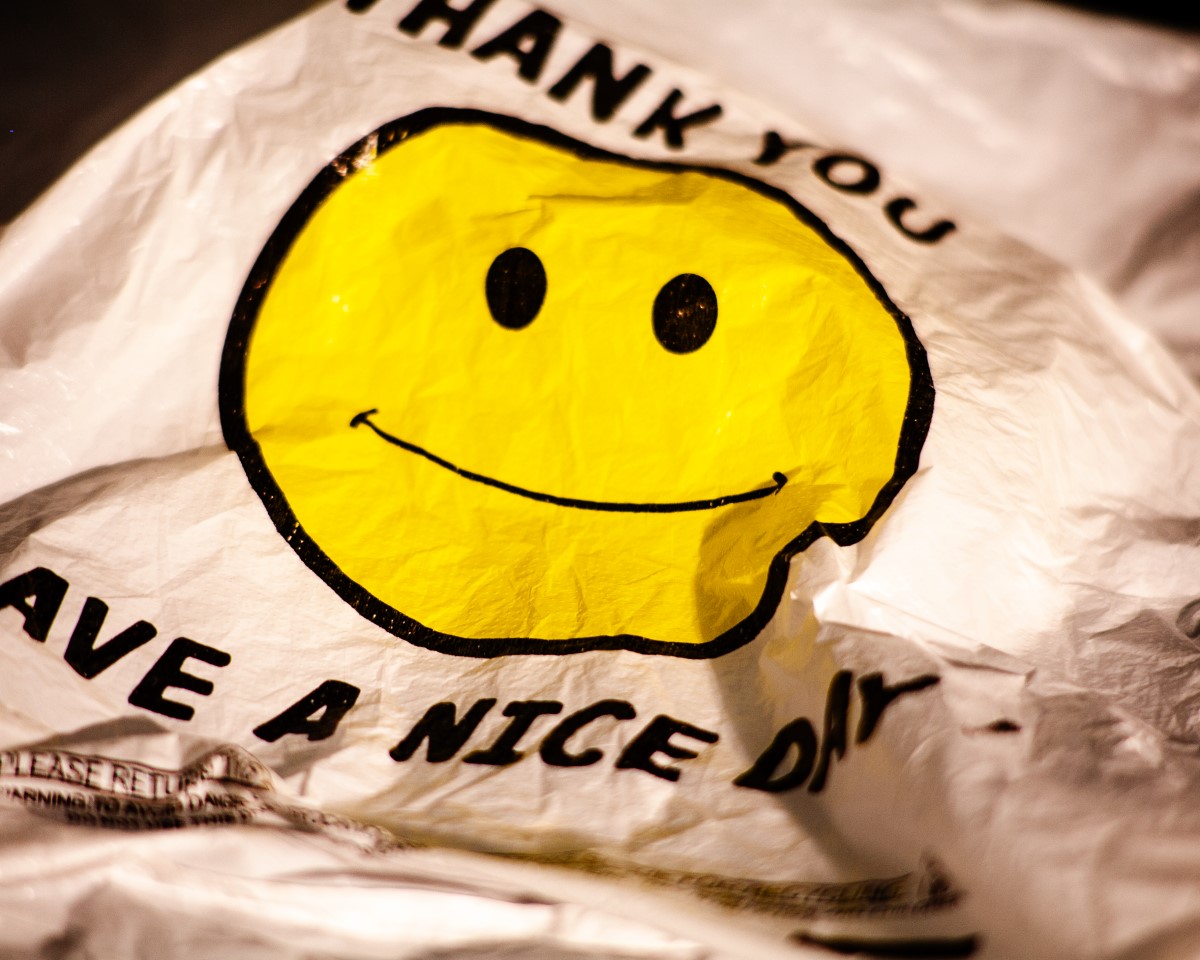
A now, uncommon, single-use plastic bag.
The world’s relationship with plastic is at a tipping point. Over the past century, plastics have revolutionized industries, making packaging lighter, cheaper, and more durable. But this convenience has come at an immense environmental cost. Every year, millions of tons of plastic waste are discarded [1], polluting oceans, clogging waterways, and taking hundreds of years to decompose [2]. The global packaging industry, responsible for a significant share of this waste, faces mounting pressure to rethink its reliance on petroleum-based plastics.
This is where bioplastics and biopolymers enter the conversation. Bioplastics refer to a diverse range of materials that are either biodegradable, biobased (derived from renewable resources), or both [3]. Biopolymers are a specific subset of bioplastics that occur naturally in living organisms, such as starch, cellulose, and proteins. Unlike conventional, petroleum-based plastics, biopolymers can break down into natural components, offering a potential solution to the environmental crisis caused by plastic pollution [4]. But while they represent a promising alternative, the path to widespread adoption is fraught with challenges.
Plastic waste, particularly from single-use items, has become a focal point of environmental policy worldwide. Governments have introduced bans, taxes, and recycling mandates to reduce reliance on conventional plastics. For example, the European Union’s single-use plastics directive [5] and Canada’s federal ban on items like straws, bags and cutlery [6] are emblematic of a broader global effort to curb plastic pollution. However, these policies often face criticism for being either too lenient or difficult to enforce. Moreover, they rarely address the underlying problem: the lack of viable alternatives that match the performance, cost, and scalability of traditional plastics.
"The fight against plastic pollution is multifaceted, involving technological innovation, policy reform, and societal behavior change. Bioplastics, particularly those made from natural biopolymers like starch and chitosan, offer a glimpse of a sustainable future. Yet, they are not a silver bullet."
Bioplastics have been hailed as a solution, but they are far from perfect. One major challenge lies in their production. Common biopolymers like polylactic acid (PLA), derived from corn starch, or polyhydroxyalkanoates (PHAs), produced by bacterial fermentation, often require significant resources, including agricultural land and energy inputs [7]. This raises questions about their overall environmental footprint compared to petroleum-based plastics. Additionally, not all bioplastics are biodegradable under natural conditions[8]. Some require industrial composting facilities, which are unavailable in many regions, leading to consumer confusion and improper disposal.
Even when biodegradable, bioplastics struggle to meet the performance standards set by conventional materials. They may lack the strength, flexibility, or moisture resistance required for common applications like food packaging [4]. This mismatch between sustainability and functionality has hindered their market penetration and forced researchers to seek innovative solutions to bridge the gap.
One promising avenue involves the use of starch to create biodegradable films. The powder you might find in your kitchen, starch, is abundant, renewable, and inherently biodegradable [9], making it a compelling alternative to conventional plastics. Starch is a polymer that can form contiguous films when exposed to sufficient heat and shear. It is known to offer excellent oxygen barrier properties [10], which are critical for preserving the freshness of packaged goods. However, starch has significant limitations, including brittleness and sensitivity to moisture [11], which restrict its application in packaging. These weaknesses highlight the challenges of relying solely on starch as a replacement for traditional plastics in demanding environments.
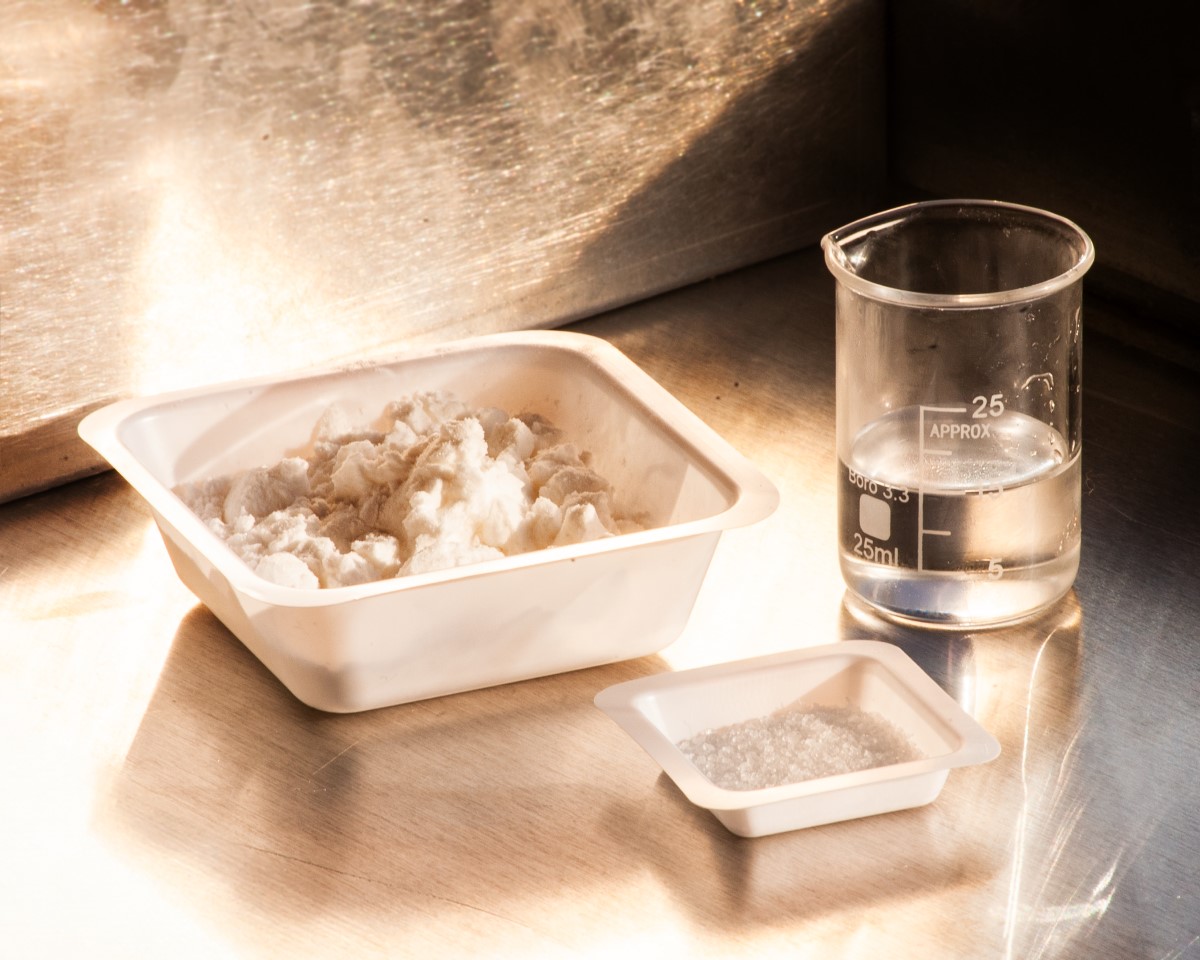
Ingredients required in the processing of Thermoplastic Starch.
To address these limitations, researchers have been exploring the potential of starch-based plastics by blending starch with other components to enhance its properties. These efforts have demonstrated that starch can be processed into thermoplastic starch (TPS), a more flexible and malleable material when combined with plasticizers like sorbitol or glycerol [11]. Such modifications help reduce starch’s brittleness and improve its usability in packaging applications. However, even with these enhancements, TPS remains susceptible to moisture, which can compromise its structural integrity and barrier performance. Despite these drawbacks, starch’s natural abundance and biodegradability position it as a strong candidate for sustainable packaging solutions [12].
One innovative strategy to improve starch-based plastics involves integrating functional additives, such as nanomaterials, to enhance their mechanical and barrier properties. Nanomaterials can create a tightly bound structure within the starch matrix, significantly improving tensile strength, elasticity, and resistance to moisture and gas permeability. These reinforcements enable starch-based plastics to better compete with traditional plastics, particularly in applications that demand durability and protection against environmental factors [13].
Current research at The Sustainable Polymers Research Lab within TMU focuses on advancing starch-based plastics by developing composite films reinforced with chitin nanocrystals (ChNCs). These nanoscale additives, derived from sustainable sources such as crustacean shells, are incorporated into thermoplastic starch matrices to address critical challenges in strength, flexibility, and moisture resistance. By leveraging the unique properties of ChNCs, this work aims to create materials that exhibit enhanced mechanical performance and superior barrier properties while maintaining biodegradability.
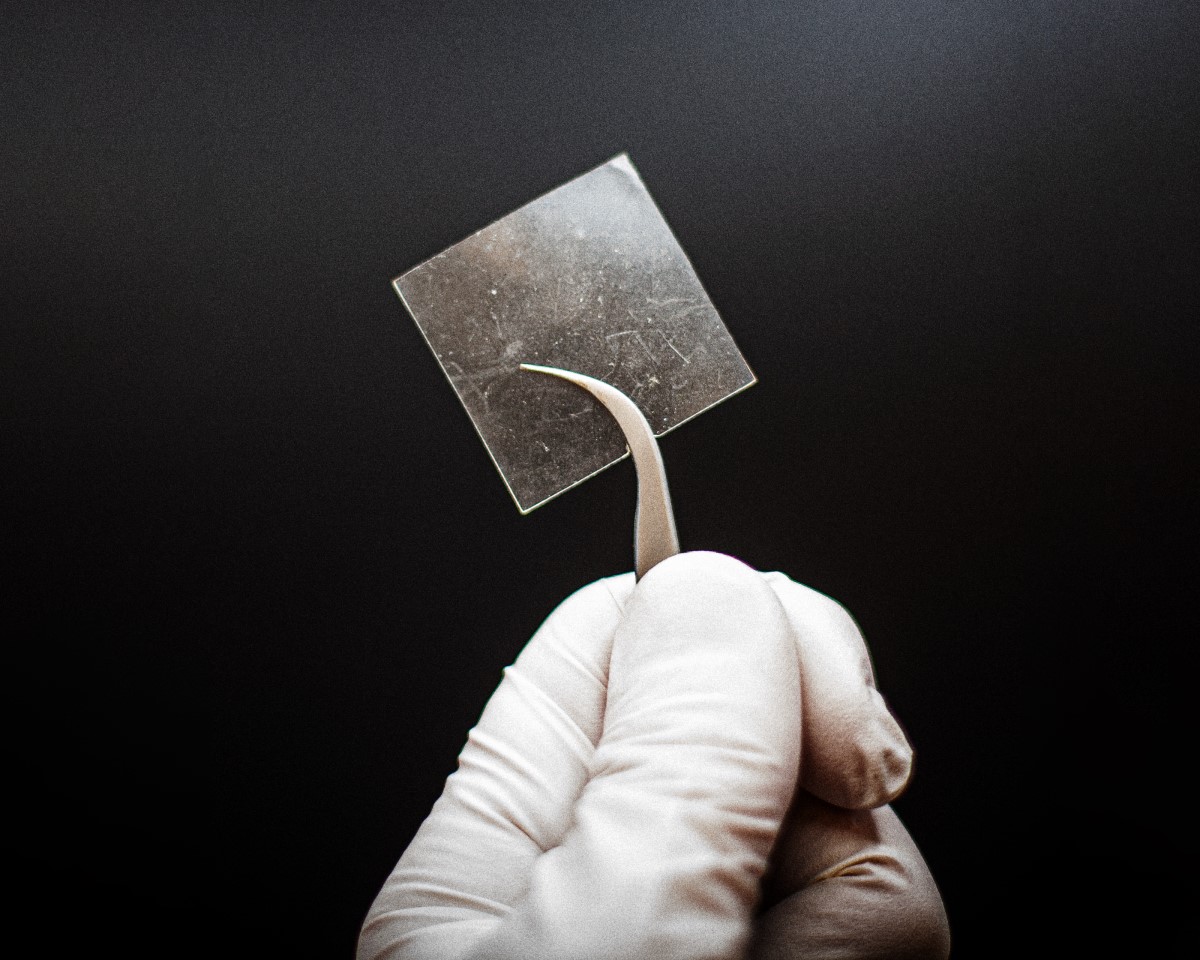
A Thermoplastic Starch film produced at the Sustainable Polymers Research Lab, TMU.
While such objectives highlight the potential of starch-based materials, they also underscore the need for further research to refine formulations and ensure consistent performance across diverse conditions.
Policy also plays a critical role in determining the success of such materials. Governments need to create a favorable environment for bioplastic innovation through subsidies, tax incentives, and funding for research and development. At the same time, regulatory frameworks must be established to ensure that new materials meet both environmental and performance standards. Without such support, the high costs and immaturity of bioplastics could deter manufacturers from adopting them, prolonging reliance on petroleum-based plastics.
Consumer awareness is another key factor. Many people remain unaware of the differences between bioplastics and conventional plastics, or the conditions required for their biodegradation. Misunderstandings can lead to improper disposal, undermining the environmental benefits of bioplastics. Clear labeling and public education campaigns are essential to ensure that consumers understand how to use and dispose of bioplastic products responsibly.
The fight against plastic pollution is multifaceted, involving technological innovation, policy reform, and societal behavior change. Bioplastics, particularly those made from natural biopolymers like starch and chitosan, offer a glimpse of a sustainable future. Yet, they are not a silver bullet. The challenges of cost, scalability, and performance must be addressed through continued research, robust policy support, and a collective commitment to environmental stewardship.
The development of starch-based plastics illustrates both the potential and the complexity of transitioning to biodegradable packaging. While the material shows promise as a sustainable alternative, its success depends on overcoming technical and economic barriers. By fostering collaboration between researchers, policymakers, and industry leaders, we can create an ecosystem that supports innovation and accelerates the shift toward a more sustainable packaging industry.
[1] The Organisation for Economic Co-operation and Development (OECD), Global Plastics Outlook. OECD, 2022. doi: 10.1787/de747aef-en.
[2] A. Chamas et al., “Degradation Rates of Plastics in the Environment,” ACS Sustain. Chem. Eng., vol. 8, no. 9, pp. 3494–3511, Mar. 2020, doi: 10.1021/ACSSUSCHEMENG.9B06635/ASSET/IMAGES/LARGE/SC9B06635_0009.JPEG.
[3] J. G. Rosenboom, R. Langer, and G. Traverso, “Bioplastics for a circular economy,” Nat. Rev. Mater. 2022 72, vol. 7, no. 2, pp. 117–137, Jan. 2022, doi: 10.1038/s41578-021-00407-8.
[4] S. Kumar, I. B. Basumatary, A. Mukherjee, and J. Dutta, “An Overview of Natural Biopolymers in Food Packaging,” Biopolym. Food Packag. Innov. Technol. Appl., pp. 1–28, Apr. 2022, doi: 10.1002/9781119702313.CH1.
[5] “Directive - 2019/904 - EN - SUP Directive - EUR-Lex.” Accessed: Nov. 18, 2024. [Online]. Available: https://eur-lex.europa.eu/eli/dir/2019/904/oj
[6] Environment and C. C. Canada, “Single-Use Plastics Prohibition Regulations: Technical Guidelines,” 2023, Environment and Climate Change Canada, Ottawa, Canada. [Online]. Available: https://publications.gc.ca/site/eng/9.927267/publication.html
[7] E. T. H. Vink, K. R. Rábago, D. A. Glassner, and P. R. Gruber, “Applications of life cycle assessment to NatureWorksTM polylactide (PLA) production,” Polym. Degrad. Stab., vol. 80, no. 3, pp. 403–419, Jan. 2003, doi: 10.1016/S0141-3910(02)00372-5.
[8] Y. Tokiwa and B. P. Calabia, “Biodegradability and biodegradation of poly(lactide),” Appl. Microbiol. Biotechnol., vol. 72, no. 2, pp. 244–251, Sep. 2006, doi: 10.1007/S00253-006-0488-1/METRICS.
[9] D. Schwartz and R. L. Whistler, “Starch,” Starch, 2009, doi: 10.1016/S1082-0132(08)X0009-3.
[10] R. Thakur, P. Pristijono, C. J. Scarlett, M. Bowyer, S. P. Singh, and Q. V. Vuong, “Starch-based films: Major factors affecting their properties,” Int. J. Biol. Macromol., vol. 132, pp. 1079–1089, Jul. 2019, doi: 10.1016/J.IJBIOMAC.2019.03.190.
[11] Y. Zhang, C. Rempel, and Q. Liu, “Thermoplastic Starch Processing and Characteristics—A Review,” Crit. Rev. Food Sci. Nutr., vol. 54, no. 10, pp. 1353–1370, 2014, doi: 10.1080/10408398.2011.636156.
[12] B. Khan, M. Bilal Khan Niazi, G. Samin, and Z. Jahan, “Thermoplastic Starch: A Possible Biodegradable Food Packaging Material—A Review,” J. Food Process Eng., vol. 40, no. 3, p. e12447, Jun. 2017, doi: 10.1111/JFPE.12447.
[13] G. Madhumitha, J. Fowsiya, S. Mohana Roopan, and V. K. Thakur, “Recent advances in starch–clay nanocomposites,” Int. J. Polym. Anal. Charact., vol. 23, no. 4, pp. 331–345, May 2018, doi: 10.1080/1023666X.2018.1447260.
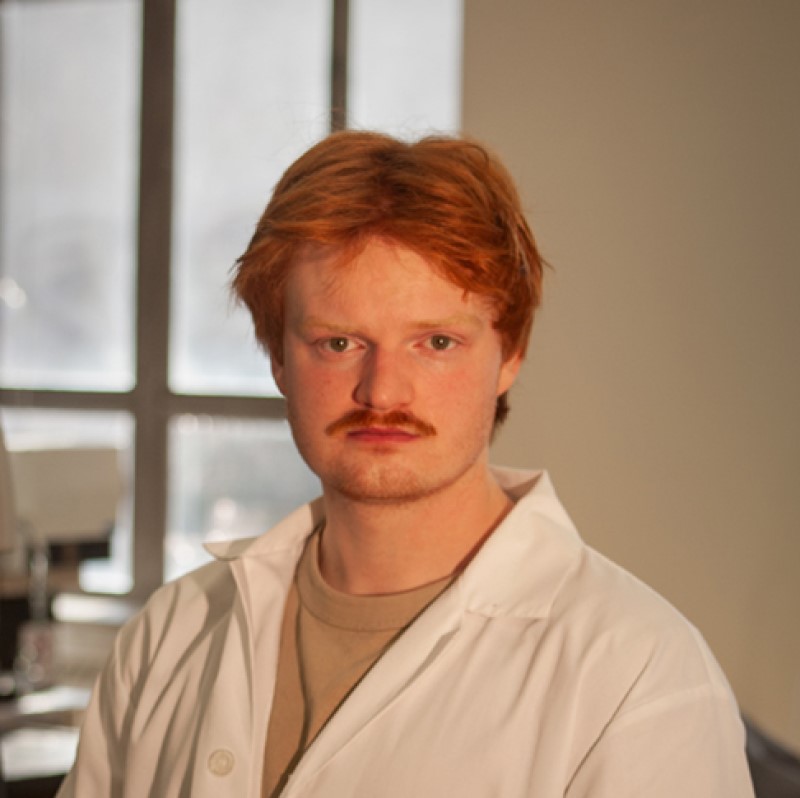
Hayden McGreal is a Master of Applied Science student in the Environmental Applied Science and Management (EnSciMan) program at Toronto Metropolitan University. Hayden is co-supervised by EnSciMan faculty members Dr. Ehsan Behzadfar and Dr. Jay Park.
Questions about the article? Contact Hayden McGreal directly at: hayden.mcgreal@torontomu.ca